The author using the test stand to measure the sound of a suppressed weapon. The microphone is on a post and the table holds the Larson-Davis meter and the laptop computer used for data recording and analysis. Photo by P.H. Dater.
By Phillip H. Dater
In the firearms sound suppression industry, we are primarily concerned with reducing exceptionally short duration, broad spectrum, impulse sounds in order to reduce their intensity and mask their characteristics. Although impartial observers can provide relatively satisfactory subjective comparison of the perceived sound signatures, the impressions will vary with the number of suppressors compared, the ability of the observers to describe the sound, and the selective hearing loss of the observer. What is pleasing to one person may be obnoxious to another, depending on hearing acuity. For example, shooters and others subjected to repeated high intensity sounds have significant hearing loss that decrease their sensitivity to high frequency sounds. In addition, typical media descriptions of suppressed sound signatures are meaningless hyperbole, describing such events as a whisper, whoosh, hiccough, or pop of a cap gun. Each of these descriptions has different connotations to different readers.
To objectively compare suppressor performance and evaluate changes during the design process, it is necessary to measure the sound level in some objective fashion utilizing standard (or consistent) conditions and precision equipment, none of which is available at Radio Shack. Reasonably accurate results require suitable equipment to measure sound pressure levels and a standardized, repeatable protocol for measurement.
All of us are familiar with the vague concept of what constitutes sound, but the specific definition is more obscure. Perhaps the simplest definition of sound is any pressure variation that the human ear can detect.
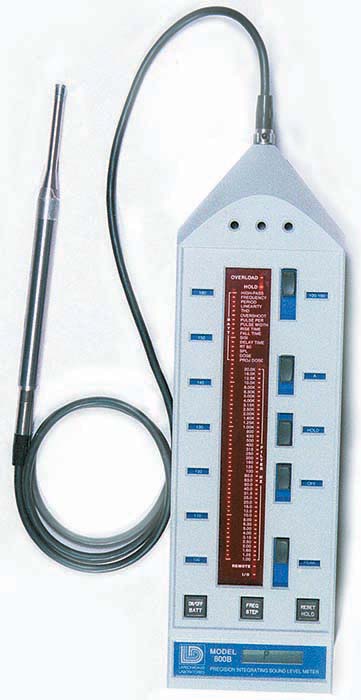
CHARACTERISTICS OF SOUND and MEASUREMENT UNIT
The number of pressure variations per second is called the frequency of the sound and is measured in Hertz (Hz). One Hertz is one cycle per second. The tone of a given sound varies with the frequency. Although certain musical notes may be a pure tone (single frequency), the vast majority of sounds in our environment are made up of multiple simultaneous frequencies.
In addition to frequency, sound is characterized by its intensity or amplitude of the pressure variations. The threshold of human hearing (minimal sound pressure) is 0.0002 dynes per square centimeter (or 20 millionths of a Pascal, abbreviated 20 (Pa). That threshold is approximately 5 billion times less than normal atmospheric pressure. This pressure variation is so small that it causes the tympanic membrane (ear drum) to move a distance less than the diameter of a hydrogen molecule. Because the ear can tolerate sound pressure levels over a million times more intense, measuring sound levels in micro-Pascals would result in some unbelievably large and unmanageable numbers. To avoid this, we use a logarithmic scale.
Logarithms are a mathematical trick to simplify the multiplication and division of unwieldy, large numbers by converting these numbers to powers of a common number, usually 10 (common or base 10 logarithms), which are the exponents of 10. Multiplication and division is then reduced to the simpler process of adding and subtracting the exponents. For example, the number 100 can be expressed as 10 squared or 10 to the 2nd power. The logarithm (abbreviated “log”) of 100 is 2. In a similar manner, the logarithm of 1,000 is 3 (10 to the 3rd power). If we were to multiply 100 by 1,000, we could simply add the exponents (or logarithms) to obtain 5 (10 to the 5th power, or 100,000). With large numbers, it is a simpler process.
The Bel (named after Alexander Graham Bell) is defined as the logarithm of the ratio of two levels of power. For convenience, we use the decibel (1/10 Bel). The decibel is not an absolute unit of measurement, and the dB scale (for sound pressure levels) uses the threshold of human hearing as the reference level, defined as 0 dB. Because of the logarithmic nature of the dB scale, each time we multiply the actual sound pressure level in micro-Pascals by a factor of 10, we add 20 to the dB sound pressure level. For example, a sound level of 200 (Pa corresponds to 20 dB; 2,000 (Pa to 40 dB; 2,000,000 (Pa to 100 dB; 200,000,000 (Pa to 140 dB; etc. The threshold of pain for impulse sounds is approximately 141 dB. Unfortunately, significant hearing damage can occur with sound levels of 140 dB where there may be no pain. Hearing can be damaged without pain or ringing in the ears!
For the purist, the term sound pressure refers to measurements made in sound pressure units (Pascals or microPascals). The term sound pressure level (SPL) refers to measurements made in decibels.
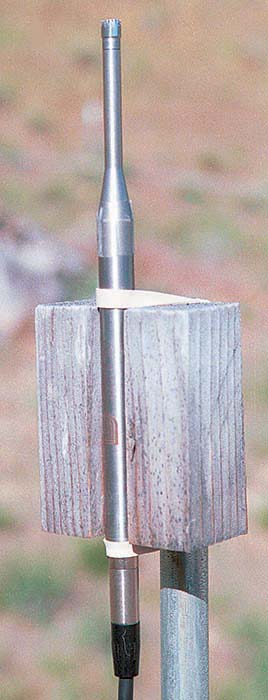
CHARACTERISTICS OF IMPULSE SOUNDS
Although most sounds in our environment are composed of relatively long duration pressure variations, there are also short and exceptionally short pressure variations referred to as impulse sounds. By definition, impulse sounds have a duration of less than one second. Because of the short duration of these sounds, the ear is less sensitive in perceiving loudness. Researchers have shown that the perceived loudness of sounds diminishes with the duration of the sound impulse. It is important to remember that although the perception of loudness is less with short impulses, the risk of hearing damage is not reduced. This will be discussed in more detail later.
Sound impulses of a firearm are generated by the sudden, explosive expansion of high-pressure gases at the moment a bullet leaves the muzzle of a firearm. The non-suppressed sound pressure level returns to normal atmospheric pressure within 100 or so microseconds of the projectile leaving the rifling. A microsecond is one one-millionth of a second. These are pretty short times. In the case of a suppressed weapon, the most intense sound pressure peak (loudest component) is an even shorter, almost instantaneous, pulse lasting at the most 20 to 25 microseconds. This is followed by several other pulses of far lower intensity for another 100-200 microseconds. If an instrument will not respond to pulse duration 20 microseconds or less, it will fail to record the loudest suppressed pulse and will provide grossly inaccurate suppressed sound levels.
HEARING DAMAGE and OSHA SPECIFICATIONS
The subject of hearing damage from high intensity sound levels is somewhat confusing with claims that sounds ranging from over 85 dB up to 140 dB or more will cause hearing damage, depending on who is making the claim. The correct answer is that all are correct, depending on conditions and characteristics of the sound. Hearing damage is dose related, whether from short duration high intensity sounds or continuous low to moderate intensity sounds. The dose is related both to the intensity of the sound and the duration (time of exposure).
If the sound were a simple on-off phenomenon (such as having a sound instantly reach full intensity and remain there until turned off suddenly, it would be fairly simple to calculate “dB-seconds,” Pascale-seconds,” or some other such measurement of sound energy over time. Unfortunately, the calculations are significantly more complex. The sound doesn’t actually start instantaneously, but rather it rises to its maximum level over a period of time and decays back to zero over a finite time interval when turned off. This makes the calculations slightly more complicated, because there is some sound dosage while the sound is building to its maximum and while it is decaying back to zero. The amount of sound dose to the ears is related to the area under the “curve” when the sound level is graphed with respect to time.
This process becomes even more complicated with real world sounds, none of which are simple on-off phenomena. What appears to be a simple pulse (as in a gunshot) is, in reality, a series of ultra short, high intensity spikes leading to the highest peak level and decaying back to near zero through a series of more spikes. Dose becomes a calculation nightmare.
Hearing damage requires a certain average dose of sound over a specified time duration. Studies have shown that whether the dose is a sustained, relatively low-level sound or whether it is a high intensity pulse makes little difference provided the absolute amount of sound energy is the same.
To help quantitate sound doses for hearing damage, the concept of L(eq) was created. L(eq) is the “equivalent noise level over a specified time interval.” Many of the figures on noise exposure are based on this figure. The L(eq) of most noises is calculated to be the equivalent of a constant sound level 40 hours a week for a full year. As an example, the sound level of a single shot from a .38 caliber revolver is approximately 156 dB. Because the duration of the noise is less than 10 milliseconds, this is the equivalent of listening to a 68 dB sound for 40 hours a week for a whole year.
The Occupational Safety and Health Administration (OSHA) has established a “legal” safe limit (Leq) of 85 dB, which is considered to be the maximum over a year’s period that does not cause hearing damage. This is called the L(eq)85, defined as a sound dose of 85 dB for 40 hours a week for one year reaching the ear. More than that causes hearing damage; less does not.
When considering gunshot noises, it is relatively simple to reasonably estimate noise dose by assuming that the duration is less than 10 milliseconds. In this estimation, which errs on the conservative side, the assumption is made that the sound instantaneously rises to the peak level and then instantaneously drops back to zero after the 10-millisecond duration. In reality, the firearm sound peak has a duration of only a few microseconds with multiple lesser peaks (still potentially harmful) lasting up to about 10 milliseconds as discussed later.
Using this model, a .22 rimfire pistol with a peak sound level of 153 dB requires approximately 115 shots to reach 85 dB L(eq). A 12-gauge shotgun at 156 dB would permit only 56 shots for the 85dB L(eq).
Hearing protection with only modest reduction (23 dB) will permit the firing of 23,000 rounds through the .22LR pistol or 11,200 rounds of 12 gauges before reaching the 85 dB L(eq). The use of a sound suppressor producing 30 dB suppression will extend the safe use of the .22LR pistol to over 115,000 rounds!
Sound measurements are made with a peak reading sound meter (described later). A peak meter records only the greatest instantaneous sound peak without consideration of the duration of the sound. When suppressing the sound at the source with a sound suppressor, there is a significant decrease in the duration (as well as energy) of the sound impulses above 85 dB. This gives a disproportionate decrease in the actual L(eq)85 per shot as compared to using hearing protectors of equivalent sound reduction.
There is a problem in that our environment is not silent, and every ambient sound over 85 dB contributes to the L(eq). Sounds assaulting our ears and eroding our permissible total dose include many sounds around our homes (lawnmower, food blender, rock music, etc.) as well as noise in the work place and in nature. Only those sounds above 85 dB really enter into consideration.
In summary, only a few high intensity pulses can be tolerated without hearing damage, while a large number of lesser intensity repetitive pulses will be classified as “safe.” Hearing protectors significantly reduce the intensity of the sound reaching the ear, permitting a greater number (or longer duration) of sounds without resultant hearing damage. Firearm sound suppressors are generally more effective at reducing the exposure than are hearing protectors, because sound suppressors attenuate the sound at the source.
The “standard” location for measuring firearm sound levels is 1 meter to the side of the muzzle. Our experience is that this location is generally close enough to the sound levels measured at the shooter’s ear for practical purposes. The next problem is how to measure firearm sounds. It is not as simple as running down to Radio Shack and getting their $40 Sound Meter.

The Larson-Davis microphone calibrator positioned on the microphone. The calibrator with its known sound level is used to confirm the accuracy of the meter system. Photo by P.H. Dater
GENERAL INSTRUMENT REQUIREMENTS
The most important specification for a sound measuring system (which includes the microphone, pre-amplifier, and meter) is referred to as the “rise time.” This is an indication of the shortest duration sound pulse that the instrument can accurately record. Although no present day transducer and system can follow the pressure rise with perfect accuracy, the device chosen must be able to reach a peak before significant pressure decay occurs. The standard instrument system for measuring the impulse sound levels of gunshots has a rise time of 20 microseconds or less as specified in MIL-STD-1474C. Meter systems with a rise time greater than 20 microseconds will miss measuring the first (and highest) suppressed impulse. Interestingly, meters with 50-microsecond rise time will usually measure a reasonably correct non-suppressed sound level because of the longer duration of the non-suppressed sound pulse. It is essential to remember that the rise time of the overall measuring system is limited by the rise time of the slowest component. If the meter is capable of a 20 microsecond rise time, but the microphone cannot respond to a pulse shorter than 100 microseconds, then the performance of the entire system is no better than 100 microseconds.
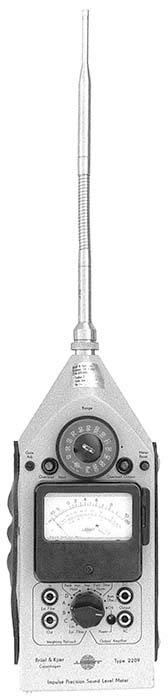
B&K Model 2209 sound level meter with microphone, adapter and microphone extension. Although long discontinued, this has been a standard of the industry for firearm sound measurements. Al Paulson photographer.
SOUND METER COMPONENTS
The basic sound level meter consists of a microphone (usually a condenser microphone) with pre-amplifier and body of the meter (which includes weighting networks, meter amplifier, detector, hold circuits, and display). Although there are a number of sound meter manufacturers, the only two producing portable meters suitable for firearm sound measurements in the field are Brüel & Kjaer (B&K) and Larson-Davis Laboratories (LDL). B&K, located in Denmark, has representatives worldwide. Larson-Davis Laboratories is located in Provo, Utah.
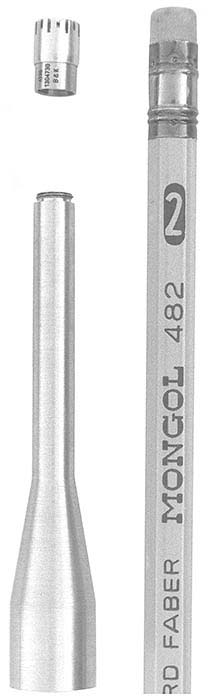
The B&K model 4136 microphone and the º inch to Ω adapter next to a No. 2 pencil for size comparison. The B&K 4136 microphone is equivalent to the Larsen-Davis 2530. Al Paulson photographer.
MICROPHONES: Types & Usage
The microphone, also referred to as a transducer, converts the sound signal to an equivalent electrical signal. Because of its stability, linearity, reliability and precision, a condenser microphone is the most suitable type of sensor for portable sound meters.
The maximum sound level is another limitation of the microphone. Remember that sound is air in motion in the form of waves of pressure. When dealing with sound levels of over 160 dB, these waves of pressure can be significant in terms of pounds per square inch. Microphones with a large diaphragm can be seriously damaged by powerful pressure waves, and the inertia of these large diaphragms can seriously limit the rise time at high sound pressure levels. For these reasons, the 1/4-inch instrumentation microphone is the standard sensor for measuring high intensity firearm sound pulses. Measuring only 1/4 inch in diameter by about 3/8 inch long, these microphones have small enough diaphragms to withstand pressures up to about 175 dB and have flat frequency responses out to around 65,000 Hz.
Although there are several types of 1/4-inch instrumentation microphones, MIL-STD-1474C specifies the use of the pressure (or random incidence) microphone for measurements. Examples of suitable pressure microphones are the B&K 4136 and Larson-Davis 2530. The pressure microphone is oriented at right angles to the sound source (usually toward the sky) so that the sound waves “graze” over the surface of the microphone. It is important to avoid subjecting any of these microphones to more than 95% relative humidity during testing, since this will affect the microphone’s calibration, frequency response and rise time.
BODY OF THE METER:
Weighting Network: Weighting is the process of altering meter sensitivity at specific frequencies to compensate for the sensitivity of the human ear at varying frequencies and sound pressure levels. Although most sound meters offer the choice of three weighting networks: “A,” “C,” and none (also called “Linear”), “A” weighting is specified by MIL-STD-1474C.
Detector: There are three basic detectors: RMS (Root Mean Square), Impulse, and Peak. The RMS and Impulse detectors have rise times varying from over 35,000 microseconds to as much as 1 second, none of which has any application in measuring firearms sound levels. Because firearm sounds are short duration pulses, and because we are interested in the absolute maximum (or peak) sound pressure generated, it is necessary to use the Peak detector. This measures the highest peak impulse that is longer than the rise time of the system.
Hold Circuit: The Hold circuit is designed to store either the maximum peak or maximum RMS sound level value. When measuring continuous sound levels, the meter will continuously update the display of the sound level. However, when measuring short duration events, the display will progress to the next event too quickly to obtain the reading. The hold circuit stores this maximum reading. For firearms sound measurements, the meter is set to utilize the peak detector and hold the result until the meter is manually reset.
Display: There are two basic types of display: analog and digital. The analog display is the conventional meter with a needle pointing to markings on a dial. In the case of the B&K 2209, the range on the dial is 10 dB without having to manually set the range. This is referred to as having a 10 dB dynamic range. Instruments that are more modern have a digital display that shows numerals on a liquid crystal display. Generally, this type of display will have a dynamic range of 60 dB without having to manually set the range.
CALIBRATORS
All electronic equipment is subject to slight changes in calibration with time and age. In order to have results that are believable, it is necessary to recheck the calibration of the sound meter, microphone, and accessories periodically. This is accomplished with a calibrator, which is an electronic device that emits a tone of a specific frequency and at a specific sound pressure level. The calibrator should be able to trace its calibration to a primary standard. In order to maintain accuracy of the system and tracability to the National Institute of Standards and Technology (N.I.S.T.), it is necessary to have the calibrator returned to the manufacturer at regular intervals (not over one year) for re-certification.
SPECIFIC METERS
There are three general categories of sound level meters: Types 0, 1, and 2. Type “0” is a laboratory reference meter and is not used for field measurements. Type “1” is classified as high precision, and Type “2” is a general-purpose meter. There probably should be another category of meters used strictly for balancing stereo systems to include those sold by Radio Shack and Edmond Scientific Co. There are two available, suitable, and reasonably affordable Type 1 measuring systems suitable for measuring the sound pressure levels of both suppressed and non-suppressed gunshots.
1. B&K 2209:
The original and traditional meter is the B&K model 2209 Impulse Precision Sound Pressure Meter, manufactured by Brüel & Kjaer. This is a portable, battery operated, relatively compact meter which was designed for field sound measurements. It uses an analog (meter needle) readout, and (in the “peak hold” mode) it will retain the highest instantaneous peak sound level. Its primary disadvantage is that it has a 10 dB dynamic range, so it is necessary to set the range selector on the meter to the correct scale. This means that the operator either must have good idea as to the sound level or else must expend a moderate amount of ammunition finding the correct range. The 2209, however, is ideal for firearm sound measurements, because it has a 20-microsecond rise time and a maximum input approaching 175 dB. B&K discontinued production of the 2209 in 1980, eventually replacing it with the 2231. The 2231 has a digital readout and wider dynamic range, eliminating the necessity of presetting the range closer than about 70 dB to the expected reading. Unfortunately, the 2231 suffers from two fatal flaws for firearm silencer measurements. It has a 50-microsecond rise time. In addition, it has a 130 dB maximum input without a separate attenuator. Furthermore, adding an attenuator may adversely affect rise time. At this time, the only instruments B&K builds with suitable rise times are measurement amplifiers, which are prohibitively expensive and require AC power, limiting their usefulness for field measurements. Fortunately, the B&K 2209 can sometimes be found on the used market, usually for about $5,000 with microphone and accessories.
2. Larson-Davis 800-B:
The second “affordable” alternative is manufactured by Larson Davis Laboratories in Provo, Utah. Similar in function to the B&K 2209, the LDL Model 800B features a digital readout with a 60 dB dynamic range. In addition, the 800B features a rise time of 20 microseconds or better. Normally, the LDL 800B has a maximum input of 140 dB, but it can be special ordered with an option to provide a 160 dB maximum input. Additionally, by recalibrating the meter to read 10 dB low, the maximum input can be stretched to 170 dB, which is more than enough for virtually all non-suppressed levels in calibers less than .50 BMG. A new meter, with the 160 dB option, 1/4-inch microphone, calibrator and accessories, will cost about $5,000.
The Larson Davis Model 800B has several interesting options. The most useful is an RS-232 interface, which allows the meter to be driven and read by a laptop computer. This can greatly speed up meter setup, data acquisition, data recording, and statistical analysis of the data. At Antares Technologies, Inc. we have written a program specifically for this purpose, which we will be happy to share with interested users.
3. Other Meters:
To the best of the author’s knowledge, there are no other readily available meters suitable for measuring firearm sound levels in the field. Inappropriate meters include the B&K 2231, the Quest 2700, and a number of other Type 1 meters. No Type 2 meters or products from Radio Shack should be even remotely considered.
ENVIRONMENTAL FACTORS AFFECTING MEASUREMENTS
Environmental factors, within reasonable limits compatible with life, affect principally the microphone and its ability to accurately translate sound variations into electrical impulses. The absorption of sound in air varies with frequency, temperature, and humidity in an exceptionally complicated fashion, and absorption is more pronounced at the higher frequencies. Besides temperature and humidity, other factors affecting sound measurement include wind, barometric pressure, and reflections from both the ground and other surfaces.
In addition, it is useful to note that firearm sound levels vary from test to test. This is because atmospheric conditions (temperature, humidity, barometric pressure, etc.) affect the burning rate of gunpowder as well as the acoustic impedance of air. Although this affects the sound levels of both suppressed and non-suppressed weapons, it is more pronounced in the suppressed weapon due to the shorter duration of the peak pulse.
Wind:
Wind has several effects on sound measurements. If over approximately 5 meters/second (12 MPH), the wind pressure will affect the movement of the microphone diaphragm, resulting in an erroneous reading. This is a particular problem with higher frequencies, especially when using a free field microphone. This problem can be minimized by using the windshield supplied with the sound meter. This shield consists of a ball of porous foam that blocks the wind pressure but has no significant attenuation of the sound pressure waves. The windshield cannot mitigate an additional wind effect. If the wind velocity is moderate, the wind will attenuate the sound upwind and reinforce the sound downwind. Both US military and NATO specify that measurements to be made when wind velocities are less than 5 meters/second.
Temperature and Atmospheric Pressure:
Increasing temperature has two effects: (1) a decrease in microphone sensitivity and (2) an increase in atmospheric absorption of sound. The microphones are rated for operation between -10 and +50 degrees Celsius (14 to 122 degrees Fahrenheit). Increased barometric pressure also decreases microphone sensitivity. However, any changes in atmospheric sound attenuation due to pressure changes are a function of temperature variations that accompany changes in barometric pressure. If necessary, peak pressure sound measurements can be scaled to standard conditions of 101.325 kPa (760mm Hg) and 288.16 degrees Kelvin (60 degrees Fahrenheit) using Sach’s Scaling Laws. Although the correction will be minimal with the temperatures and pressures involved, it is necessary to record both temperature and ambient (station) atmospheric pressure if scaling is anticipated.
Humidity:
High humidity slightly decreases absorption of sound by the atmosphere. All of the condenser measurement microphones will operate in a satisfactory manner when the relative humidity is between 5 and 95 percent. More than 95 percent humidity may cause moisture condensation on the microphone diaphragm, which will significantly decrease microphone sensitivity.
Ground Surface Effect & Reflections:
As mentioned previously, the inverse square law states that the sound intensity decreases with the square of the distance. Because a reflected path from the noise source to the microphone is always a greater distance than the direct path through the air, it is logical to assume that peak sound intensity from the reflected path will always be less than that from the direct path and will have no effect on the maximum peak reading. Experiments in Finland, however, have shown empirically that this is not necessarily true in the case of suppressed measurements, and that measurements made over concrete will be approximately 2+ dB higher than measurements made over grass or other sound-absorbing material. This suggests that the maximum peak of the suppressed pulse is less than 20 microseconds in length, and the reflected pulse with its slight extra delay is being measured. Regardless, US Military and NATO standards dictate that measurements are made over a non-reflecting surface, such as grass.
MEASUREMENT TECHNIQUES and a SUGGESTED MEASUREMENT PROTOCOL
Authorities in the measurement of overpressure associated with explosions suggest that sound pressure measuring systems featuring a 20 microsecond rise time (such as the B&K 2209 or LDL 800B) will miss some of the initial peak. Although this may be true, the bias is probably small (in the order of one or two decibels). In spite of this small bias, the standard of the firearms suppressor industry, NATO, and the US military is to utilize instrumentation with a 20-microsecond rise time.
Under ideal circumstances, the investigator would locate the weapon and transducer (microphone) in a temperature-controlled, echo-free environment with no operator in the measurement field. As with a lot of ideal scenarios, this is impractical, and some compromises must be tolerated.
Measurements are standardized as being made one meter (39.34 inches) 90 degrees to the side of the muzzle and at a height of 1.6 meters (5 feet) above ground over grass or another non-reflecting surface. The process is simplified if the microphone and preamplifier can be separated from the meter with a cord. In this situation, the simplest microphone stand is to insert a 6-foot piece of conduit or rebar a foot into the ground and then attach the microphone and preamplifier to the pole at the 5-foot level with rubber bands. A folding table back a few feet can hold the meter and accessories. If the microphone cannot be located remotely from the meter, the meter can be mounted in place using a tall camera tripod or a camera clamp attached to the post.
Spacing of the firearm from the microphone is also critical, and the most basic method, suggested by Al Paulson, is to tie a one-meter piece of dental floss to the microphone. The other end of the dental floss is tied to a rubber band that loops over the muzzle of the weapon. A piece of brightly colored string attached to nails can be laid out on the ground in a right angle to help maintain consistent alignment of the weapon visually. Far more sophisticated setups can be constructed.
The next step in obtaining sound measurements after setting up the jig or fixture is meter calibration. The meters rarely drift, and calibration should remain constant. However, to be certain that the equipment is functioning normally, it is necessary to check the sound meter system with a calibrator that has been recertified within the past 12 months. Each meter uses slightly different calibration procedures. For the LDL 800B, the meter is set to utilize the fast detector with no weighting (linear). After placing the calibrator on the microphone, the meter is checked and adjusted for a reading of 114 dB according to instructions by Larson Davis.
For the firearm sound measurement, the meter is set to utilize the peak detector in the “hold” mode (to freeze the highest peak reading), and the weighting is set to “A” (as specified in MIL-STD-1474C).
Normally, non-suppressed readings are made first, primarily because if changes in the setup need to be made, it will not be necessary to repeat all of the suppressed readings to keep all data consistent. If the non-suppressed reading on the LDL 800B is greater than 160.5 dB (the meter maximum input), it will be necessary to either change the microphone spacing or, preferably, recalibrate the instrument to read 10 dB low and add 10 dB to each reading.
Just prior to each measurement, press the reset on the meter to clear the memory and ready it for the next sound pulse. Hold the weapon parallel to the ground at shoulder height (1.6 meters) with the muzzle in such a position as to make it perpendicular to the microphone. The string on the ground visually helps in this alignment. Move the weapon sideways just until the slack is out of the piece of dental floss and the rubber band is not stretched. Fire the weapon. After making the weapon safe, record the meter reading, reset the meter, and repeat the process. After 10 rounds, average the data to provide the mean non-suppressed sound level.
Using the same procedure, repeat the 10 measurements with the suppressor attached to the weapon and average this data to obtain the mean suppressed level. The difference between these means is the degree of suppression. When recording the data, be certain to also record the ambient temperature, humidity, barometric pressure, and date. Although you should record the temperature with a thermometer at the location of the tests, the other data can be obtained from the nearest weather bureau or from NOAA weather broadcasts, which are available throughout the United States. It is important to record the station pressure (actual atmospheric pressure in the vicinity of the test site), not the pressure corrected to sea level. There are some excellent and affordable digital barometers, hydrometers and thermometers available that will allow the investigator to make on-site measurements of the required data.
The last step is to recheck the meter calibration with the calibrator to be certain that there has been no meter drift. There should be none, and the recheck calibration should be within 0.1 dB of the initial calibration. If there has been a greater change, there are several possible interpretations. (1) Check battery condition and replace if necessary. (2) Verify that environmental conditions do not exceed the operating specifications of all equipment being used. (3) If neither of the preceding checks has revealed the problem, then return the meter, calibrator and microphone to the manufacturer for necessary repairs and recalibration and recertification.
With all sound measurements, it is important to remember that there will be minor day-to-day variations in the data obtained, which appear primarily to be due to ambient temperature variations. Such variations will commonly be a decibel or two.
SOURCES
B&K has numerous sales representatives throughout the world. They can be contacted at Brüel & Kjaer Instruments, Inc., 185 Forest St., Marlborough, MA 01752; phone (508) 481-7000. Although the B&K 2209 is no longer in production, some of the factory representatives can occasionally help locate a used instrument. B&K also has some measurement amplifiers that are well suited for measuring suppressed gunshots, but the cost of a complete setup will be in the tens of thousands of dollars. B&K can also direct users to providers of short-term leases or rentals, although this is an expensive option for more than a month or two.
Larson Davis Laboratories has sales representatives throughout the United States. LDL can be contacted directly at 1681 West 820 North, Provo, UT 84601, phone (801) 375-0177. For the calibrator, 1/4-inch pressure microphone, and microphone extension cable, the current cost is about $5,000. The RS-232 computer interface will add another $850.
Although not involved in the sale or supply of sound measuring equipment, Antares Technologies Inc. (Box 3538, Boise, ID 83703) has developed software to drive the Larson Davis 800B from a DOS-based laptop computer. This software sets and resets the meter, records the data on disk, and performs statistical analysis on the data.
References:
Brüel & Kjaer, Measuring Sound, 1984
Brüel & Kjaer, Measurement Microphones, 1988
Brüel & Kjaer, Sound Intensity, 1988
Crum, Richard (FBI) and Owen, Edward (BATF), “Silencer Testing,” Crime Laboratory Digest, v14n2, April 1987
Department of Defense, Military Standard Noise Limits for Military Materiel (Metric), MIL-STD-1474C, 8 March 1991
Grubelich, Mark, Sandia National Laboratories (Albuquerque, NM), personal communication (1990)
International Organization for Standardization, Acoustics Testing of silencers in situ, ISO/TC 43/SC 1, 1994
North Atlantic Council (NATO) Defense Research Group, Panel on the Defense Applications of Human and Bio-Medical Sciences Research Study Group on the Effects of Impulse Noise, AC/243(Panel 8/RSG.6), 6 April 1987
Paulson, Alan C., personal correspondence
Sampson, Steven, “A Decibel Primer,” 73 Magazine, July 1995
This article first appeared in Small Arms Review V3N11 (August 2000) |