By Alton P. Chiu
Conventional wisdom trades barrel weight for accuracy and precision. Proof Research combines these attributes in its composite carbon fiber and 416R barrel. Through interview with Proof Research and the author’s own rudimentary math, this piece briefly explores the physical and thermal properties affecting accuracy and precision, as well as how Proof Research mitigates these concerns. Performance was evaluated over a 65-round string using a 1:8 twist, 16-inch AR-15 barrel with an intermediate gas length (PN 101599).
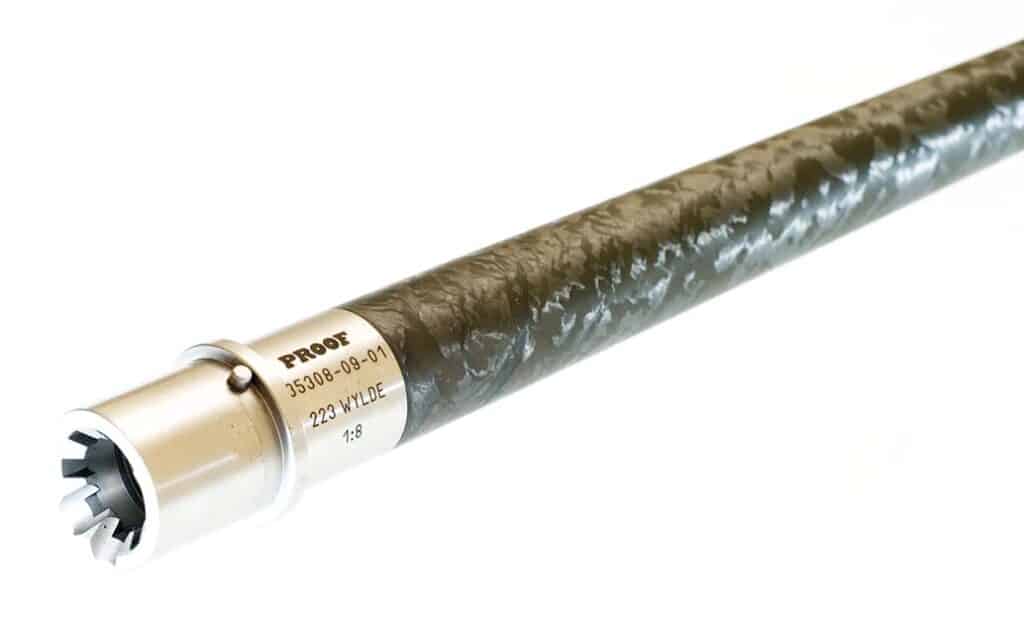
Barrel Harmonics
An aphorism goes that all models are wrong, but some are useful. This section develops a wrong but useful “back-of-the-napkin” model to illustrate the vibration characteristics between lightweight and tar-get profile steel barrels. Generally speaking, less vibrations yield better accuracy, but the exact relationship is a complex subject requiring proprietary knowledge not available to the author.
Firing a cartridge forms an impulse event that spreads energy across the frequency domain and excites all structural modes. A free-floated barrel, with uniform profile and material, can be modeled as a uniform cantilever beam. The fundamental frequencies can be written as follows:
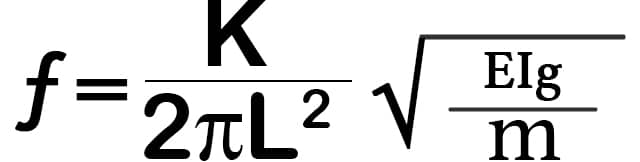
f is the frequencies (Hz), K is a constant with values 3.52 for primary, 22 for secondary and 61.7 for tertiary mode. L is the beam length (m), E is Young’s modulus (Pa), g is acceleration due to gravity (m/s2), and m is mass per unit length (kg/m). I is the area moment of inertia (m4) computed as equation 2.
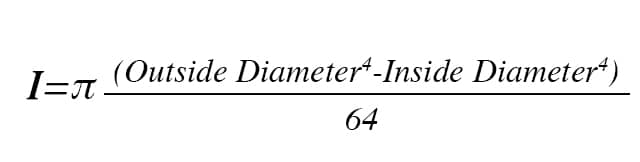
Assume 4150 steel with a nominal 200 Gpa, 16-inch barrel length, 0.224-inch inside diameter. For lightweight profile, assume 0.625-inch uniform outside diameter. For target profile, assume 0.936-inch uni-form outside diameter. The vibration frequencies can be computed as follows.
One could compute the pure bending tip deflection from frequency using the relationship below.
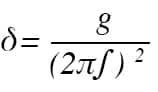
Lightweight Profile | Target Profile | |
---|---|---|
Primary Mode (Hz) | 60 | 104 |
Secondary Mode (Hz) | 376 | 648 |
Tertiary Mode (Hz) | 1054 | 1817 |
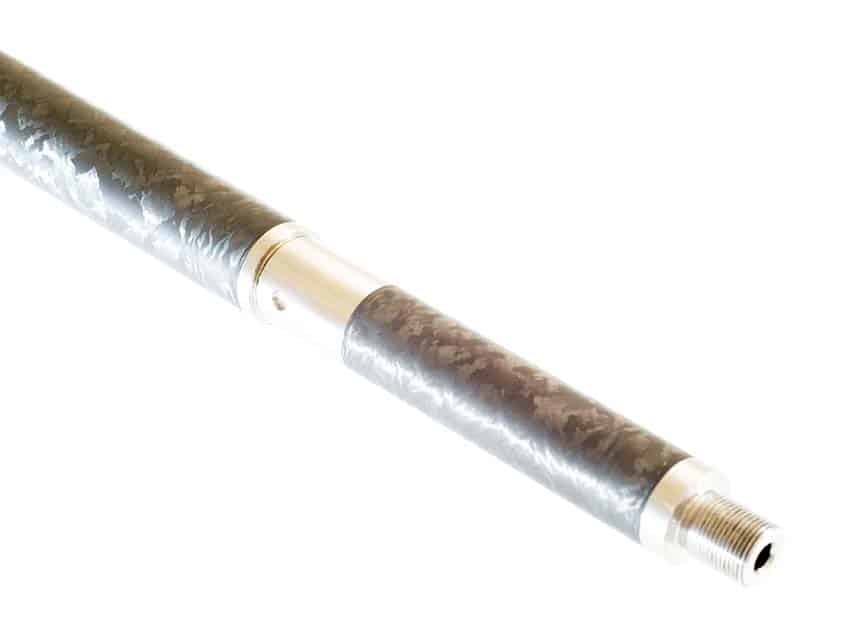
but the results would be disproportionately small to real-world deflection observed in slow-motion video footage. This is because the bar-rel experiences tension (recoil and Newton’s First Law of Motion: The Law of Inertia), torsion (imparting bullet spin and Newton’s Third Law of Motion: For every action there is an equal and opposite reaction), bending (gravity), as well as axial and hoop stress (gas pressure). Thus, it moves in more of a circular cone motion than mere whipping in a vertical plane. These complex interactions are best solved with Finite Element Analysis not available to the author.
One can still grasp the relationship between barrel profile and harmonics using these equations. A thicker profile increases the area moment of inertia, making it stiffer. This increases fundamental frequencies and reduces deflections, agreeing with the conventional wisdom that one trades weight for accuracy and precision. A Proof engineer indicates that while this generally results in better accuracy (ceteris paribus), there are many other factors and interactions. It is important to note that this relationship is not directly applicable to comparing a Proof composite barrel against a similarly weighted steel barrel, as their Young’s modulus differ.
Proof composite barrels are made of reduced contour 416R steel, then helically wrapped with continuous fibers on a computer-controlled winder. The company claims “the aerospace-grade carbon fiber we use has a specific strength up to 30 times that of stainless steel and a specific stiffness up to 7 times greater than steel” (Proof Research. (2019). Composite Rifle—Proof Research. [online] Available at: proofresearch.com/the-science/ [Accessed 14 Mar. 2019]). The author found this statement insufficient for “back-of-the-napkin” performance comparisons. First, specific strength is force per unit area at failure divided by density (Nm/kg). Since forces experienced at firing should not approach
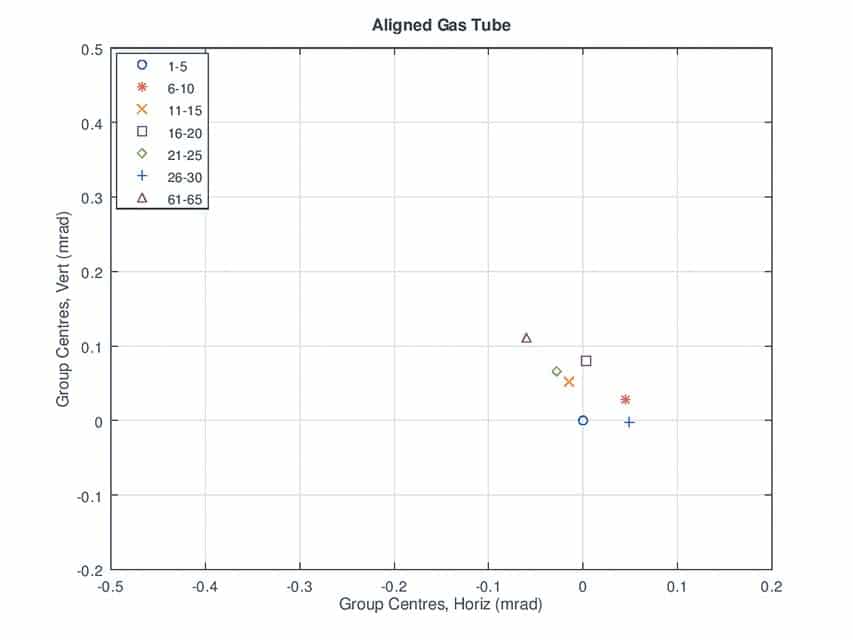
failure, this metric is not directly applicable but merely hints at the improvement. Second, specific stiffness is stiffness (force divided by deflection) to weight ratio, and the statement specified neither the mode (e.g., tension or torsion or bending) nor dimension. Note the nominal density difference between carbon fiber (1.6 g/cm3) and steel (7.7 g/cm3) already accounts for a factor of 4.4. Lastly, the composite barrel has an unknown internal profile that precludes any reasonable computation. The author had requested but not received more information from Proof Research due to the proprietary nature of these details.
At this point, the reader may be frustrated with the qualifications and ultimate inability to provide a clear and direct comparison between steel and composite barrels. The author promises recompense by way of an unequivocal demonstration in the shooting section.
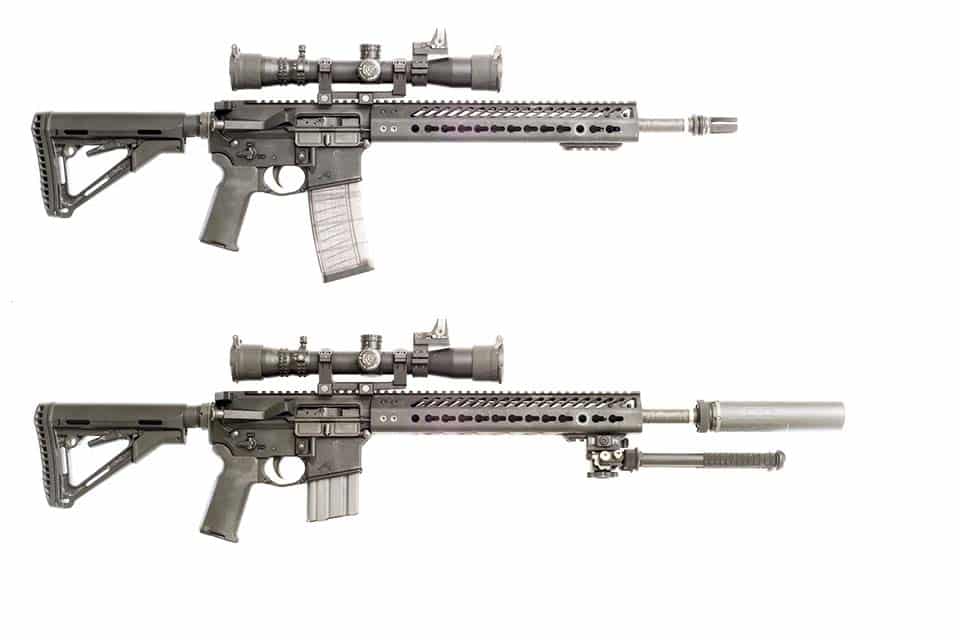
Thermal Effects
This section examines the detrimental effects of heat over a long string of fire, and how Proof both mitigates and delays that. The vibrational characteristics previously discussed could be considered constant across the working temperature of a barrel, as both Young’s modulus and dimensional changes are minor.
On the other hand, manufacturing inadequacies can degrade accuracy and precision as the barrel inevitably heats up. Bore dimension changes can cause varying bullet start. Residual stress from rifling can deflect the barrel. To mitigate this, Proof uses 416R steel ordered from foundry to spec, then performs all other steps (e.g., cutting, rifling, lap-ping, etc.) in house so as to maintain tight tolerances. Proof Research claims a very stress-free process that results in uniform and repeatable bore and groove as foundational elements to accuracy. The same process is used on both composite and steel barrels.
Another mitigation is to expel heat quicker, so as to delay the onset of degradation. Although the barrel conducts heat into the receiver, the contact area is the same between different barrels’ profiles and is thus omitted from this discussion. Consider the free convection transfer rate in equation 4 and the black body radiative transfer rate into vacuum in equation 5,

where q is transfer rate, A is barrel surface area, Tb is barrel surface temperature, and Ta is ambient temperature. One can deduce that a larger surface area (i.e., thick profile barrel) cools faster.
Another consideration is the efficiency in transporting heat from where it is deposited (chamber and bore) to where it is dissipated (surface), a small pot. Heat transfer from the case wall and gasses to the chamber and bore are conductive and forced convective respectively. Their rates depend upon bore temperature, which differs between barrel profiles due to their different cooling rate, making a study of temperature versus round count difficult.

Proof Research promotes surface cooling with a thick profile while speeding the transport of heat from the chamber to the surface with a carefully designed helical fiber wrapping pattern. Because the chamber end of the barrel experiences hot gasses longer, its surface temperature 1/2×28 threads on the muzzle. Gas block area comes dimpled.
is higher than the muzzle end. The fibers diffuse heat along the length of the barrel to even it out and promote cooling. Furthermore, the carbon fiber and resin are designed to expand at the same rate as steel, so as to prevent de-lamination and point-of-impact shift. Although Proof declined to provide test data, it assured the author various contours of steel and composite barrels were tested in order to substantiate its claim of faster cooling.
Lastly, one should be reminded that factors external to barrel (e.g., receiver or stock contact point) can also result in thermal-in-reduced, point-of-impact shift. For example, M1 Garand match modifications ensure the upper handguard (clipped to barrel) does not contact the stock while the front handguard (attached to lower band which pulls against the stock) should not contact the barrel (Roberts Jr., J.B. “Accurizing the M1 Rifle.” The M1 Rifle, An Ameri-can Rifleman Reprint: 16. Print). As detailed in the shooting section below, the author discovered contact between his AR-15 gas key and gas tube, and this likely induced vertical stringing and lateral group shift.
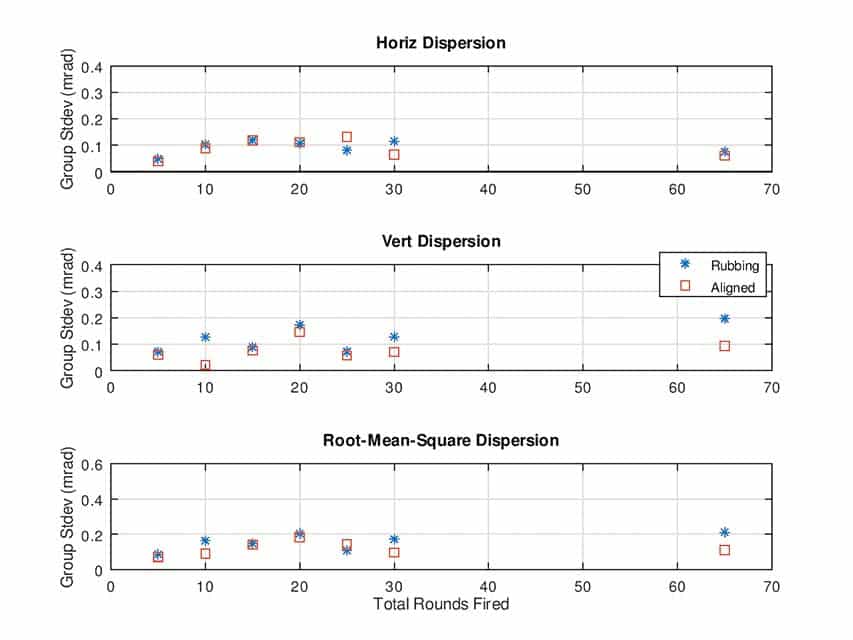
Test AR-15 Barrel
Since the claims of Proof Research are difficult to substantiate with rudimentary models, the improvements must be observed in action. After all, the proof of the pudding is in the eating. The bar-rel chosen is a 16-inch, 1:8 twist, intermediate gas length composite barrel with a .223 Wylde chamber. Intermediate gas system is 1.5 inches shorter than a rifle-length system and longer than a mid-length one. Not being standard, a gas tube is also supplied. The gas port journal measuring 0.75-inch diameter is fully stainless steel and is dimpled for a set screw gas block; although the author utilized a clamp type adjustable gas block.
Proof Research offers both steel and composite barrels because light-ness is not universally desired. For carrying across mountains or burning through a close-quarters stage, low weight affords comfort and agility. After all, an old joke goes that an M1 Garand weighs 9.8 pounds with the decimal point falling out somewhere along a road march. Opposite is true for precision (e.g., PRS) rifles where weight increases polar moment of inertia and steadies an unstable shooting position such as off-hand or barricade. Author found the composite barrel to be a good compromise between steadiness and agility. For action matches, the rifle felt quick-to-hand even with multiple optics attached. For PRS matches, forward weight from a bipod and suppressor added steadiness.
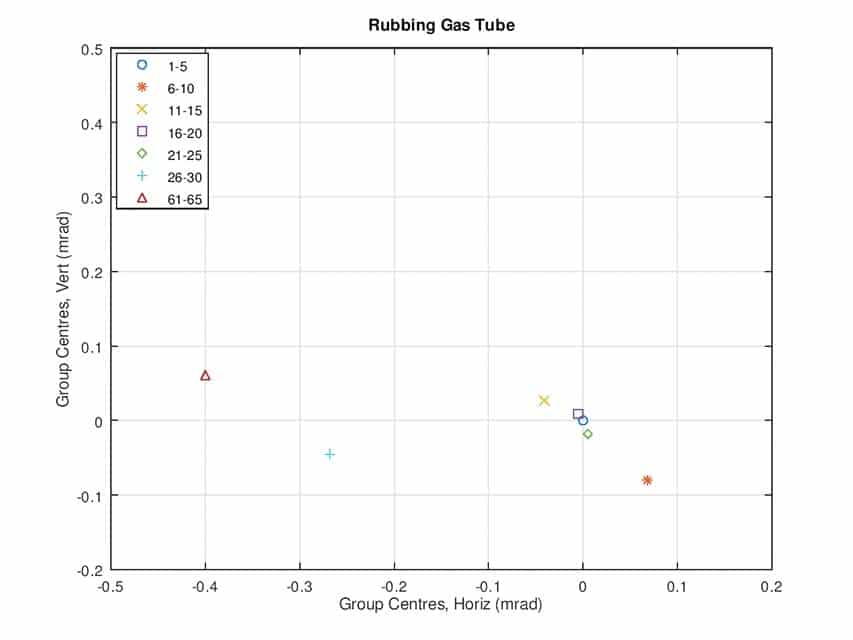
Test Procedure
To evaluate both accuracy and precision over a long string of fire, the first 30 rounds were fired in five-round groups. These illustrated performance typically seen by most users. Thirty rounds were then fired in quick succession to generate heat, and the test concluded with five rounds fired in a group in order to highlight thermal effects.
The author fired from a prone position supported by the bipod and rear bag with a 10x scope at a 50m distant target. The human element was consciously added to observe mirage effects and to verify if the shifts were buried within discernible difference. The author fired at a cadence reserved for rapid fire portion of NRA high-power rifle matches, roughly one shot every four seconds. A pace of one round per second was used for the 30-round “mag dump.” There were magazine changes between each of the five-round groups, but the bolt was closed to minimize chamber convective cooling.

Results and Discussion
A peculiar lateral left shift and vertical stringing were noted when the test was first conducted. This was likely linked to heat as the effect showed in both rounds 26-30 group and 61-65 group. With the help of a Proof Research engineer, gas tube to gas key contact on the left and upper portions (looking from chamber to muzzle) was identified as the likely culprit. This data set is titled, “Rubbing Gas Tube” below. The clamp-type gas block was realigned and the gas tube slightly tweaked so the bolt carrier group slides home without shifting or resistance. The test was repeated and data presented with the title, “Aligned Gas Tube.” Considering the first 30 shots, the Proof barrel exhibited remarkably good accuracy and precision. Screening out the 26-30 group with the rubbing gas tube, both datasets exhibited point-of-impact shift within 0.2 Miliradian (MRAD) in horizontal and vertical directions. With a standard deviation of 0.1 to 0.2 MRAD, one cannot conclude the shift to be statistically significant. Putting it plainly, the author reasonably expects the first 30 shots to be within a 0.2 MRAD area. Note that although the adjusted gas tube data showed more shifts, it is likely due to the author committing shooting position errors.
Group centers for rounds 61-65 in both datasets are interesting. Lateral offset in the rubbing dataset is likely due to a rifle installation problem and not indicative of barrel performance. After correction, this group still showed an up-shift which is likely due to a barrel heat mirage. The author noted a heavy and boiling mirage developed during the 30-round “mag dump” and persisted into this last group. This mirage carried the target image high, causing the user to aim high, and, consequently, bullet impacts were high. The lateral offset is not likely a mirage effect but a position or natural point of aim error due to user fatigue. There were baffles on either side of the rifle to block wind. The gas tube adjustment also shrank vertical dispersion in this last group to be in line with the rest.
Infrared thermometer data was recorded on both the Proof Research composite and steel lightweight barrels but not presented in this piece owing to difficulty in meaningful comparisons. Chiefly, it was impossible to maintain the same timing of shots, ambient temperature or radiative heat from the sun. Infrared thermometer measures thermal radiation and is calibrated on a certain emissivity value. Differing barrel material has differing emissivity and does not allow for a direct comparison. Thus, this data did not add value to this discussion.
Conclusion
Proof Research’s composite barrel is a happy combination of light weight, accuracy and precision. Meticulous and stress-free manufacturing, combined with carbon fiber engineered for superior heat dissipation, produced an accurate and precise barrel even through a long string of fire. Although direct comparisons between composite and steel barrels proved difficult without the necessary but proprietary data, a shooting test unequivocally demonstrated its accuracy and precision. Despite carrying a hefty price tag (MSRP $920 USD), the author found the composite barrel a very worthwhile addition on his modern sporting rifle used for both precision and “run-and-gun” tasks.
This article first appeared in Small Arms Review V23N7 (AUG/SEPT 2019) |